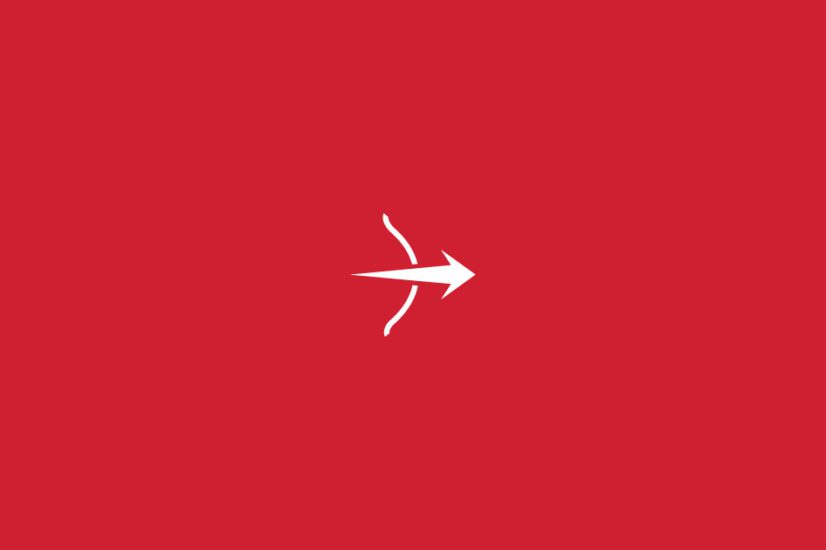
Posted March 2, 2010
In this issue of The Lancet Neurology, Rinne and colleagues1 report something of a breakthrough by demonstrating the feasibility of eventually testing the so-called amyloid hypothesis of sporadic Alzheimer’s disease in vivo. According to their analysis, a passive immunotherapy protocol with an amyloid-β monoclonal antibody (bapineuzumab) was associated with a decrease in the cerebral PET signal after administration of the amyloid plaque imaging compound carbon-11-labelled Pittsburgh compound B (11C-PiB). This finding of a change in PiB signal over time is exciting, and the interpretation favoured by the authors is that the new results suggest that bapineuzumab has increased the clearance of cerebral amyloid β. This is a logical conclusion drawn from the dramatic changes in cerebral amyloid burden that have been consistently found in amyloid precursor protein transgenic mice after either active or passive immunotherapy.2 Although other explanations are possible (eg, perhaps bapineuzumab-coated amyloid β binds PiB poorly), the authors’ conclusion is the most sensible in view of the results of the post- mortem study by Nicoll and colleagues,3 in which there was surprisingly low plaque density
in the cerebral cortices of patients with Alzheimer’s disease who had received amyloid-β immunisation in an earlier active immunisation trial.
At least two anti-amyloid clinical trials (of homotaurine and tarenflurbil) have been reported as failures in modifying the course of mild-to-moderate Alzheimer’s disease.4,5 However, in neither of these trials were amyloid biomarkers used as endpoints. This would be the equivalent of a statin trial in which myocardial infarction was recorded as an endpoint but plasma cholesterol was not even measured. No trial can be said to be a test of the amyloid hypothesis unless CSF amyloid-β concentrations or cerebral amyloid burdens are documented and quantified.
Conversely, the recent trial of bapineuzumab reported by Salloway and colleagues6 and the study by Rinne and colleagues1 reported little or no obvious clinical response to bapineuzumab. So-called amyloid naysayers might seize on this as proof that anti-amyloid therapy should be abandoned. This would be an irresponsible and irrational response. Mutations or polymorphisms in at least four genes, each on different chromosomes, have been shown to cause or dramatically increase the risk for the Alzheimer’s phenotype.7 Each of the mutations fulfils Koch’s postulates for causing or enhancing amyloid pathology in mouse models.8 Mice do not develop a phenotype of cerebral amyloidosis except in the presence of human amyloid β in the context of a pathogenic Alzheimer’s mutation or a risk factor polymorphism.9 These are compelling, unchangeable facts suggesting involvement of amyloid β in genetic forms of Alzheimer’s disease.
In about 97% of patients with Alzheimer’s disease, no pathogenic mutation can be identified.10 APOEε4, SORL1, and CLU, among others, have been associated with risk of developing sporadic Alzheimer’s disease.11 It is probably not a coincidence that each of these genes can also be linked to amyloid-β metabolism in cell biology experiments.12 However, the naysayers have a point when they favour the notion that, in common sporadic Alzheimer’s disease, metabolic disturbances (perhaps involving calcium or oxidative stress) upstream of amyloid-β deposition might cause some of the neurodegeneration directly and independently of amyloid β.7 This argument can be supported with known molecular pathways,13,14 but, so far, not with pathogenic mutations. Still, logic dictates that amyloid β cannot be causative and toxic in genetic forms of the disease and yet totally harmless and irrelevant in common sporadic forms. The only way to settle the issue is to prevent amyloid-β accumulation by intervening, guided by biomarkers, at a presymptomatic age (probably in the fourth or fifth decade of life), proving with serial biomarker measurements that the intervention prevented amyloidosis; then long- term assessment (ie, until 80 or 90 years of age) with neuropsychological testing could verify whether the amyloid-β-free brain is still destined for failure.
Some subtleties are yet to be accounted for. After a century of focusing on amyloid plaques, attention has recently shifted to the less well-defined amyloid-β oligomers as the key proximate neurotoxins in Alzheimer’s disease.15 Moreover, PiB binds only to fibrillar amyloid β and not to oligomeric amyloid β, so there is, as yet, no way to visualise or quantify the cerebral burden of oligomeric amyloid β. The nature of the interaction between bapineuzumab and oligomeric amyloid β is unknown. If oligomeric amyloid β is truly the key toxin, then whatever prophylaxis is used must also rid the brain of the oligomeric species.
Notably, also on the horizon in Alzheimer’s treatment is latrepirdine, a retired Russian antihistamine with surprising apparent benefit in both Alzheimer’s disease and Huntington’s disease.16 The mechanisms of action of latrepirdine are poorly understood but they are rapidly becoming a focus of great interest. New information suggests that the mechanisms might involve the protein aggregation phenomena that are involved in both diseases. Latrepirdine modulates amyloid-β metabolism in vivo in amyloid precursor protein transgenic mice17 and accelerates clearance of protein deposits from synuclein transgenic mice.18
Although it is premature to say that we have effective, disease-modifying drugs available, these emerging data concerning both bapineuzumab and latrepirdine move us closer to the goal of understanding, treating, and, eventually, preventing major neurodegenerative diseases such as Alzheimer’s disease.
Sam Gandy
Mount Sinai School of Medicine, Departments of Psychiatry and Neurology, New York, NY 10029, USA [email protected]
I have received grants from the Forest Research Institute and from Amicus Pharmaceuticals, and I am a consultant to Diagenic and a member of the Safety Monitoring Committee for the Elan and Johnson & Johnson AAC-001 trial. Research in my laboratories includes work on latrepirdine, but I receive no financial support from Pfizer or Medivation.
1 Rinne JO, Brooks DJ, Rossor MN, et al. Pittsburgh compound B PET assessment of amyloid-β load in patients with Alzheimer’s disease treated with bapineuzumab: a phase 2, double-blind, placebo-controlled, ascending-dose study. Lancet Neurol 2010; published online XXXXX. DOI: XXXXX [LT: add info when available]
2 Lemere CA, Masliah E. Can Alzheimer disease be prevented by amyloid-beta immunotherapy? Nat Rev Neurol 2010; 6: 108–19.
3 Nicoll JA, Barton E, Boche D, et al. Abeta species removal after Abeta42 immunization. J Neuropathol Exp Neurol 2006; 65: 1040–48.
4 Swanoski MT. Homotaurine: a failed drug for Alzheimer’s disease and now a nutraceutical for memory protection. Am J Health Syst Pharm 2009; 66: 1950–53.
5 Green RC, Schneider LS, Amato DA, et al; Tarenflurbil phase 3 study group. Effect of tarenflurbil on cognitive decline and activities of daily living in patients with mild Alzheimer disease: a randomized controlled trial. JAMA 2009; 302: 2557–64.
6 Salloway S, Sperking R, Gilman S, et al; Bapineuzumab 201 clinical trial investigators. A phase 2 multiple ascending dose trial of bapineuzumab in mild to moderate Alzheimer disease. Neurology 2009; 73: 2061–70.
7 Gandy S. The role of cerebral amyloid beta accumulation in common forms of Alzheimer disease. J Clin Invest 2005; 115: 1121–29.
8 Tanzi RE, Bertram L. Twenty years of the Alzheimer’s disease amyloid hypothesis: a genetic perspective. Cell 2005; 120: 545–55.
9 Bush AI, Pettingell WH, Multhaup G, et al. Rapid induction of Alzheimer A beta amyloid formation by zinc. Science 1994; 265: 1464–67.
10 Bertram L, Tanzi RE. The genetic epidemiology of neurodegenerative disease. J Clin Invest 2005; 115: 1449–57.
11 Sleegers K, Lambert JC, Bertram L, Cruts M, Amouyel P, Van Broeckhoven C. The pursuit of susceptibility genes for Alzheimer’s disease: progress and prospects. Trends Genet 2010; 26: 84–93.
12 Small SA, Gandy S. Sorting through the cell biology of Alzheimer’s disease: intracellular pathways to pathogenesis. Neuron 2006; 52: 15–31.
13 Bezprozvanny I, Mattson MP. Neuronal calcium mishandling and the pathogenesis of Alzheimer’s disease. Trends Neurosci 2008; 31: 454–63.
14 Zhu A, Raina AK, Perry G, Smith MA. Alzheimer’s disease: the two-hit hypothesis. Lancet Neurol 2004; 3: 219–26.
15 Lublin AL, Gandy S. Amyloid-beta oligomers: possible roles as key neurotoxins in Alzheimer’s disease. Mt Sinai J Med 2010; 77: 43–49.
16 Sabbagh MN, Shill HA. Latrepirdine, a potential novel treatment for Alzheimer’s disease and Huntington’s chorea. Curr Opin Investig Drugs 2010;11: 80–91.
17 Steele JW, Kin SH, Cirrito JR, et al. Acute dosing of latrepirdine (Dimebon ),TM a possible Alzheimer therapeutic, elevates extracellular amyloid-beta levels in vitro and in vivo. Mol Neurodegener 2009; 4: 51.
18 Bachurin SO, Ustyugov AA, Peters O, Shelkovnikova TA, Buchman VL, Ninkina NN. Hindering of proteinopathy-induced neurodegeneration as a new mechanism of action for neuroprotectors and cognition-enhancing compounds. Doklady Biochemistry and Biophysics 2009; 428: 235–38.
19 Aslund A, Sigurdson CJ, Klingstedt T, et al. Novel pentameric thiophene derivatives for in vitro and in vivo optical imaging of a plethora of protein aggregates in cerebral amyloidoses. ACS Chem Biol 2009; 4: 673–84.